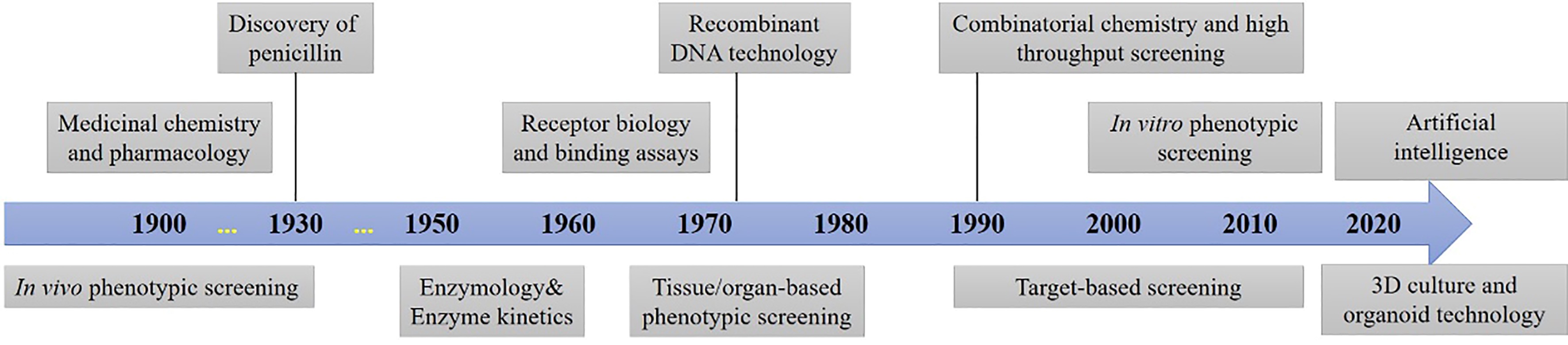
Downloads
Download


This work is licensed under a Creative Commons Attribution 4.0 International License.
Review
Advances of the Target-Based and Phenotypic Screenings and Strategies in Drug Discovery
Shoubao Wang 1,*, Zihan Wang1, Lianhua Fang1, Yang Lv2, and Guanhua Du1,*
1 Beijing Key Laboratory of Drug Targets Identification and Drug Screening, Institute of Materia Medica, Chinese Academy of Medical Sciences & Peking Union Medical College, Beijing, 100050, China.
2 Beijing Key Laboratory of Polymorphic Drugs, Institute of Materia Medica, Chinese Academy of Medical Sciences & Peking Union Medical College, Beijing, 100050, China.
* Correspondence: shoubaowang@imm.ac.cn (Shoubao Wang); dugh@imm.ac.cn (Guanhua Du).
Received: 8 November 2022
Accepted: 10 December 2022
Published: 21 December 2022
Abstract: Drug discovery and development is a complex and expensive process. There are two approaches, phenotypic and target-based approaches, each holding different advantages for screening novel drug candidates when pursuing successful marketing authorization. However, the attrition rates of drug candidates continue to increase. In this review, we discuss recent successes and ongoing advances in phenotypic screening and target-based screening for drug discovery. We also explore how strategic and technological innovations may fuel new approaches in drug discovery. There are two approaches in drug discovery.
Keywords:
drug discovery phenotypic approach target-based approach screening1. Introduction
Drug discovery and development is one of the most complex scientific practices that also embraces cooperation between multiple disciplines. Modern drug discovery can be traced back to the end of the nineteenth century with great advances in experimental biology and medical research, as well as in organic chemistry [1].
Looking through the history of drug discovery, there are two distinguishable strategies, the phenotypic approach and the target-based approach. The phenotypic approach is a target-agnostic approach based on the observations of drug effect on disease. The first 100 years of modern drug discovery were largely phenotypic drug discovery [2].
Over the past three decades, drug discovery has shifted away from in vivo/in situ phenotypic approach, and towards target-based strategies. The target-based approach is based on a rational approach, generally starting with target identification and screening of drug candidates based on known targets [3]. It is efficient and is widely used for drug discovery with the advancement of high throughput screening technologies.
However, for complex diseases, most drugs are highly ineffective, and the success rate of drug candidates has been steadily declining. Moreover, in drug discovery, two serious problems, target deconvolution and polypharmacology, cannot be solved by the phenotypic and target-based approaches [4]. This article reviews the evolution and advances of the two strategies in drug discovery. The inherent limitations with phenotypic and target-based approaches are also analyzed to determine if there are approaches to address them. The purpose of this review is to find out if there are innovations may fuel the next generation approaches in drug discovery.
Figure 1. Evolution of modern drug discovery.
2. Target-Based Screening (TBS) in Drug Discovery
In the target-based approach, a target is often a single gene, gene product or molecular mechanism associated with the disease of interest identified from basic research. After an appropriate target is validated and a suitable assay is developed, target-based screening is conducted. G-protein-coupled receptors (GPCRs), ion channels and enzymes represent the most common and successful targets for drug discovery. Once a hit compound is discovered and optimized for better activity, its efficacy should be confirmed in the cellular or organismic level.
The target-based approach emerged along with advances in pharmacology and chemistry in the early 20th century. It was further developed with molecular biology and genomic science in the late 1980s [5]. Over the past decades, target-based drug discovery has become the main paradigm used in early drug discovery, and brought an increased screening capacity and the rise of rational drug discovery. It was believed that target-based screening has some distinct advantages over phenotypic screening and would result in an increased productivity.
3. Biochemical Assays in Target-Based Screening
Biochemical assay generally utilizes a purified target protein of interest and measures the change of the protein function in vitro, for instance, enzymatic activity. These assays are generally conducted in competition format, in which the compound under study must displace a known ligand or substrate. The wealth and depth of studies performed in the 1950s and 1960s on enzymes and enzyme kinetics provided a method for precise calculation of compound’s potency (IC50 or EC50) and efficacy (% maximal response). Hundreds of enzymes were discovered and purified during this period, later becoming important molecular targets of drug discovery [5]. These assays are typically conducted in 96-, 384- and 1536-well plates with an optical method such as absorbance, fluorescence, or luminescence that provide a balanced choice among assay volumes, throughput, costing, and sensitivity [6,7].
4. Biophysical Assays in Target-Based Screening
Binding-based assays check directly at the compounds binding to the protein of interest, independent of their effects on the protein function. In general, binding-based assays use biophysical techniques that cover a series of techniques such as microscopy, spectroscopy, and molecular modelling. The biophysical assays study the structure, properties and dynamics of biomolecules at an atomic or molecular level, contrasting with the biochemical methods where the alteration of function or the inhibition of probe/protein binding is measured. The fragment-based drug design (FBDD) is one particularly suitable application for biophysical methods. The methods commonly used in FBDD are nuclear magnetic resonance (NMR), surface plasmon resonance, and X-ray crystallography [6,8].
5. Advances in Target Identification for Drug Discovery
Over the past decade, in spite of increasing levels of investments, there has been a steady decline in the number of new drugs that enter the market. A striking observation, however, is that the decline in productivity has, to a large extent, coincided with the introduction of target-based drug discovery [3]. Although the lack of drug efficacy in late-stage drug development can be the result of multiple factors, including poor correlation of animal models with human diseases and genetic variation of patient populations, invalidated targets for disease is a significant factor for many failed drug candidates [5,9]. Too few targets have been identified that meet the criteria for having both disease relevance and acceptable safety. Due to new advances in human cell-based basic research combined with breakthroughs in molecular biology, genomics, proteomics, and computational biology, understanding target biology and drug mechanism-of-action within a disease-relevant context is becoming realistic with the exponential growth of molecular data.
6. Technical Advances for Target-Based Screening
On the basis of traditional fluorescent methods, some new useful fluorescence-detection approaches including fluorescence anisotropy/polarization (FA/FP), fluorescent resonance energy transfer (FRET), fluorescence lifetime analysis (FLA) and AlphaScreen (Amplified Luminescent Proximity Homogeneous Assay) were developed and applied in high-throughput screening [6,10].
In terms of binding-based methods, several high-throughput formats such as surface plasmon resonance (SPR), differential scanning fluorimetry (DSF, also known as thermal shift assay, TSA), DNA-Encoded Library technology (DELs) are available for high-throughput screening. DELs are built by a ‘split-and-pool’ method in which chemical scaffolds are mixed together and then separated into vessels for the next round of chemistry [11].
7. Polypharmacology and Network Pharmacology for Drug Discovery
Traditionally, drugs have been designed with the aim of targeting a single biological entity, usually a protein (the so-called “on-target”), with high selectivity to avoid unwanted effects arising from mis-targeting other biological targets (“off-targets”) [12]. The “one disease-one target-one drug” dogma obstruct innovations and has been transformed into “one drug multiple targets”, known as polypharmacology. Polypharmacology is emerging as the next paradigm of drug discovery [13]. It can be applied to identify new off-targets and is particularly important for prediction of possible adverse effects of new drugs in development[14]. On the other hand, it can also be used for drug repurposing where new indications of existing drugs can be identified [13,14]. This will significantly improve the currently stalling drug discovery engine.
Figure 2. There are two distinguishable strategies in drug discovery: phenotypic approach and target-based approach.
Network pharmacology embraces the complexity of disease and supports the development of rational drug combinations and polypharmacology strategies at earlier phases in the drug discovery, therefore promoting the shift of the paradigm from a “one-target, one-drug” mode to a “network, multi-target therapeutic” mode [15].
8. Phenotypic Screening (PS) in Drug Discovery
Phenotypic screening is a paradigm used in drug discovery to identify molecules with the ability to alter a phenotype in cells, tissues or an entire organism. The term ‘phenotype’ juxtaposed with ‘genotype’ lacks clear definition, relying on semantic description, for example, morphology, behavior, appearance, structure, etc [16]. Therefore, early phenotypic screenings were largely conducted empirically, assisted by serendipity and astute observation.
Phenotype-based approach is not a new approach and has re-emerged as an alternative platform of drug discovery following a comparison of the discovery strategies for new molecular entities (NMEs) approved by the U.S. Food and Drug Administration (FDA) between 1999 and 2008 [17,18]. In contrast to target-based screening, phenotypic screening does not rely on knowing the identity of the specific target in the disease. In phenotype-based approach, the phenotype associated with the disease of interest is first selected. The phenotype-based assay platform is constructed, leading to hit compound discovery and further hit optimization for modulating the designated phenotype. After a hit compound is screened out, a target identification study should be performed to understand its mechanism-of-action. A key advantage of phenotypic screening over target-based screening is the capacity to capture complex biological mechanisms that are not otherwise achievable.
9. Human/Animal-Based in Vivo Phenotypic Screening
In vivo evaluation is a key element in phenotypic screening, as in vivo model systems can provide enriched information in addition to valuable efficacy and generally offer a hit a higher chance of success. Historically, human or animal models were involved in phenotypic screening. They were used to discover new drugs by experience or drug repurposing. Interesting examples are the use of Aspirin and the repurposing of Sildenafil. In addition, an in vivo system contributes to the evaluation of multiple signaling mechanisms and inter-tissue crosstalks that is not available with a cell-based system. This is still the only platform available for safety and efficacy studies with a higher chance of biomedical applicability to human use [19].
A variety of animal models are available for phenotypic screening, models as simple as Saccharomyces cerevisiae or Dictyostelium discoideum, invertebrates such as Artemia salina, Drosophila melanogaster (fly) and Caenorhabditis elegans (worms), lower vertebrates such as Danio rerio (zebrafish) and Oryzias latipes (medaka fish), Xenopus leavis, Gallus gallus, to mammalian models such as Tupaia belangeri, Mus musculus and Rattus norvegicus [19]. In the past decades, the screening capacity of several small animals including Caenorhabditis elegans, Drosophila melanogaster, Xenopus laevis, and zebra fish has been improved to a relatively high screening throughput by using 96-well plates, although it is still a significantly lower throughput compared to cell-based assays [5].
10. Cell-Based (In Vitro) Assays
Cell-based (in vitro) assays are defined as any assay that takes place within a living cell. They can be grouped into categories such as cell proliferation assays, cell death assays, reporter gene assays, and cell signaling assays. One of their significant advantages is the ability to be easily adapted to a high-throughput format along with high automation. Another key feature of cell-based screening is that multiple targets can be screened at once, the readout being the outcome of a cellular pathway or network. Functional readouts can also provide enriched information about transmembrane permeability and cytotoxicity that may not be gained in the biochemical and binding assays. Heterogeneity can also be measured, particularly if cells are visualized by high-content imaging.
With technical advances in microscopy, imaging, image analysis software, automation and array of in vitro and in vivo models, the throughput of cell-based screening has been greatly improved, allowing the re-emergence of phenotypic screening to be an attractive platform for drug discovery over the past ten years. Furthermore, numerous state-of-the-art methods such as flow cytometry, laser scanning fluorescence, microfluidics and electrophysiology methodologies are readily available for conducting high-throughput phenotypic screening [19].
11. Next-Generation Phenotypic Screening
Although there has been substantial development in phenotypic screening, there are still challenges associated with this approach. Firstly, cell lines widely used in phenotypic screening rarely originate from human beings, let alone patients, and thus lack the disease-specific genetic background. The culture conditions for phenotypic screening assays differ markedly from the physiological environment of the diseased tissue, where cells interact and communicate with both each other and the extracellular matrix. Finally, validation of screening hits, mechanism-of-action profiling and uncovering the molecular targets of these hits, also known as target deconvolution, are also important issues [20].
Fortunately, several approaches have been employed to help overcome these challenges and optimize phenotypic screening as a potent approach to next-generation drug discovery.
12. Application of Human Induced Pluripotent Stem Cells (iPSCs)
In vitro cell cultures are crucial research tools for modeling human development and diseases. The fundamental interspecies differences make it impossible for common cell lines to accurately mirror or fully recapitulate human clinical pathophysiology. It is also difficult to extend findings on efficacy and toxicity testing from other species to humans [21,22]. In recent years, human iPSCs have emerged as an attractive platform for overcoming these conventional limitations of non-human models for disease modeling and drug discovery. Because iPSCs can be differentiated into any type of disease-relevant cells and can faithfully recreate the genetic background of patients, iPSC models have been widely used to study monogenic diseases as well as more complex polygenic diseases of various organs. With the establishment of the differentiation protocol of human iPSCs into the cell types of interest, the differentiated cells from human iPSCs have garnered more attention in drug screening.
12. Three-Dimensional (3D) Culture and Organoid Technologies
Although the conventional monolayer cell cultures have been widely used in the past, the lack of tissue architecture and complexity of such model fails to inform the true biological processes in vivo. Recent advances in 3D culture and organoid technology have revolutionized the in vitro culture techniques. Today, organoids derived from either adult stem cells or pluripotent stem cells can be developed to resemble various organs [23]. Many types of organoids, such as intestinal organoids, brain organoids, liver organoids, skin organoids, and kidney organoids, are beginning to be used in medical research. Organoid models recapitulate the cellular heterogeneity, structure, and functions of their original organs and hold great promise in disease modeling [24].
Recently, organ-on-a-chip (OOC) systems that are based on microfluidic technology and 3D culture technologies have emerged to accurately simulate the physiologically relevant conditions and functionalities of organs [25]. Besides mimicking individual organs, advancements of OOC systems provide evidence for the fabrication of “multi-organ-on-chip” (MOC) models, which is ideal for monitoring the complex interactions between multiple organs [25,26].
13. Artificial Intelligence for Phenotypic Screening
Artificial intelligence (AI) is a branch of computer science that aims to mimic the recognition, learning, perceiving, problem-solving and decision-making capabilities of the human mind. Much of the previous skepticism against usage of AI in pharmaceutical drug discovery are fading, consequently benefitting the pharmaceutical industry. AI techniques have inspired computer-aided drug discovery. AI can recognize hit and lead compounds and provide a quicker validation of the drug target and a better understanding of drug-target interactions. Algorithms, such as Nearest-Neighbour classifiers, random forest (RF), extreme learning machines, support vector machines (SVMs), and deep neural networks (DNNs), are already used for predicting in vivo activity and toxicity [27].
AI-aided approaches have only begun to address some fundamental problems in drug discovery. Certain methodological advances, open data sharing and model development will likely help address some of the most challenging questions and play a central role in drug discovery [28].
14. Concluding Remarks
Despite considerable progress, phenotypic screening and target-based screening often share a same conceptual challenge: the highly streamlined assay biology may fail to recapitulate signaling pathways and physiological traits of disease-relevant cell types in vivo, and assay results may not translate well into human clinical outcomes [29].
Given the complexity in screening, it can be expected that next-generation screening approaches will complement rather than replace conventional phenotypic screening and target-based screening. The sweet spot might be an integrated paradigm in which phenotypic approach and target-based approach are used in conjunction with each other in the discovery of drugs. This move towards combining the two approaches has been enabled by advances in technology that can more subtly manipulate molecular processes in cells.
To accomplish this, Hiroaki Iwata and his colleagues developed a new in silico method using the probabilistic framework. This method is based on a machine learning technique that integrates data from compound-target protein interactions obtained from the target-based approach and data from compound-phenotype associations obtained from the phenotypic approach. This method integrates both the phenotypic and target-based approaches to estimate a relevant network from compound to phenotype [30].
Today, it is becoming clear that the convergence of phenotypic-based and target-based methods is more important than their “either/or” comparison [31]. The ambition and hope are that the combined targeted phenotypic approach integrated with appropriate biological model systems will evolve more effective strategies and innovative drugs in diseases that are yet to have a cure.
Author Contributions: Conceptualization, Guanhua Du; writing—original draft preparation, Shoubao Wang and Zihan Wang; writing—review and editing, Lianhua Fang; visualization, Zihan Wang; funding acquisition, Yang Lv and Shoubao Wang. All authors have read and agreed to the published version of the manuscript.
Funding: This research was funded by the Chinese Academy of Medical Sciences (CAMS) Innovation Fund for Medical Sciences (CIFMS), grant numbers 2022-I2M-2-002 and 2022-I2M-1-015.
Conflicts of Interest: The authors declare no conflict of interest.
References
- Eder J.; Herrling P.L. Trends in modern drug discovery. Handb. Exp. Pharmacol., 2016, 232: 3-22. DOI: https://doi.org/10.1007/164_2015_20
- Moffat J.G.; Vincent F.; Lee J.A.; et al. Opportunities and challenges in phenotypic drug discovery: an industry perspective. Nat. Rev. Drug Discovery, 2017, 16(8): 531-543. DOI: https://doi.org/10.1038/nrd.2017.111
- Sams-Dodd F. Target-based drug discovery: is something wrong? Drug Discov. Today. 2005, 10(2): 139-147. DOI: https://doi.org/10.1016/S1359-6446(04)03316-1
- Hurko O. Target-based drug discovery, genetic diseases, and biologics. Neurochem. Int., 2012, 61(6): 892-898. DOI: https://doi.org/10.1016/j.neuint.2012.01.016
- Zheng W.; Thorne N.; McKew J.C. Phenotypic screens as a renewed approach for drug discovery. Drug Discovery Today, 2013, 18(21/22): 1067-1073. DOI: https://doi.org/10.1016/j.drudis.2013.07.001
- Blay V.; Tolani B.; Ho S.P.; et al. High-throughput screening: today's biochemical and cell-based approaches. Drug Discov. Today., 2020, 25(10): 1807-1821. DOI: https://doi.org/10.1016/j.drudis.2020.07.024
- Stoddart L.A.; White C.W.; Nguyen K.; et al. Fluorescence- and bioluminescence-based approaches to study GPCR ligand binding. Br. J. Pharmacol., 2016, 173(20): 3028-3037. DOI: https://doi.org/10.1111/bph.13316
- Togre N.S; Vargas A.M.; Bhargavi G.;et al. Fragment-based drug discovery against mycobacteria: the success and challenges. Int. J. Mol. Sci., 2022, 23(18): 10669. DOI: https://doi.org/10.3390/ijms231810669
- Parasrampuria D.A.; Benet L.Z.; Sharma A. Why drugs fail in late stages of development: case study analyses from the last decade and recommendations. AAPS J., 2018, 20(3): 46. DOI: https://doi.org/10.1208/s12248-018-0204-y
- Yasgar A.; Jadhav A.; Simeonov A.; et al. AlphaScreen-based assays: ultra-high-throughput screening for small-molecule inhibitors of challenging enzymes and protein-protein interactions. Methods Mol. Biol., 2016, 1439: 77-98. DOI: https://doi.org/10.1007/978-1-4939-3673-1_5
- Zhao G.X.; Huang Y.R.; Zhou Y.; et al. Future challenges with DNA-encoded chemical libraries in the drug discovery domain. Expert Opin. Drug Discovery, 2019, 14(8): 735-753. DOI: https://doi.org/10.1080/17460441.2019.1614559
- Ramsay R.R.; Popovic-Nikolic M.R.; Nikolic K.; et al. A perspective on multi-target drug discovery and design for complex diseases. Clin. Transl. Med., 2018, 7(1): 3. DOI: https://doi.org/10.1186/s40169-017-0181-2
- Reddy A.S.; Zhang S.X. Polypharmacology: drug discovery for the future. Expert Rev. Clin. Pharmacol., 2013, 6(1): 41-47. DOI: https://doi.org/10.1586/ecp.12.74
- Nováček V.; Mohamed S.K. Predicting polypharmacy side-effects using knowledge graph embeddings. AMIA Joint Summits on Translational Science proceedings. AMIA Jt. Summits Transl. Sci. Proc., 2020, 2020: 449-458.
- Hughes R.E.; Elliott R.J.R.; Dawson J.C.; et al. High-content phenotypic and pathway profiling to advance drug discovery in diseases of unmet need. Cell Chem. Biol., 2021, 28(3): 338-355. DOI: https://doi.org/10.1016/j.chembiol.2021.02.015
- Mahner M.; Kary M. What exactly are genomes, genotypes and phenotypes? And what about phenomes?. J. Theor. Biol., 1997, 186(1): 55-63. DOI: https://doi.org/10.1006/jtbi.1996.0335
- Swinney D.C.; Anthony J. How were new medicines discovered? Nat. Rev. Drug Discovery, 2011, 10(7): 507-519. DOI: https://doi.org/10.1038/nrd3480
- Swinney D.C.; Lee J.A. Recent advances in phenotypic drug discovery [version 1; peer review: 2 approved]. F1000Research, 2020, 9(Faculty Rev): 944. DOI: https://doi.org/10.12688/f1000research.25813.1
- Szabo M.; Svensson Akusjärvi S.; Saxena A.; et al. Cell and small animal models for phenotypic drug discovery. Drug Des., Dev. Ther., 2017, 11: 1957-1967. DOI: https://doi.org/10.2147/DDDT.S129447
- Vincent F.; Nueda A.; Lee J.; et al. Phenotypic drug discovery: recent successes, lessons learned and new directions. Nat. Rev. Drug Discovery, 2022, 21(12): 899-914. DOI: https://doi.org/10.1038/s41573-022-00472-w
- Liu C.; Oikonomopoulos A.; Sayed N.; et al. Modeling human diseases with induced pluripotent stem cells: from 2D to 3D and beyond. Development, 2018, 145(5): dev156166. DOI: https://doi.org/10.1242/dev.156166
- Sayed N.; Liu C.; Wu J.C. Translation of human-induced pluripotent stem cells: from clinical trial in a dish to precision medicine. J. Am. Coll. Cardiol., 2016, 67(18): 2161-2176. DOI: https://doi.org/10.1016/j.jacc.2016.01.083
- Corrò C.; Novellasdemunt L.; Li V.S.W. A brief history of organoids. Am. J. Physiol.: Cell Physiol., 2020, 319(1): C151-C165. DOI: https://doi.org/10.1152/ajpcell.00120.2020
- Takahashi T. Organoids for drug discovery and personalized medicine. Annu. Rev. Pharmacol. Toxicol., 2019, 59: 447-462. DOI: https://doi.org/10.1146/annurev-pharmtox-010818-021108
- Zhao Y.; Kankala R.K.; Wang S.B.; et al. Multi-organs-on-chips: towards long-term biomedical investigations. Molecules, 2019, 24(4): 675. DOI: https://doi.org/10.3390/molecules24040675
- Ronaldson-Bouchard K.; Teles D.; Yeager K.; et al. A multi-organ chip with matured tissue niches linked by vascular flow. Nat. Biomed. Eng., 2022, 6(4): 351-371. DOI: https://doi.org/10.1038/s41551-022-00882-6
- Paul D.; Sanap G.; Shenoy S.; et al. Artificial intelligence in drug discovery and development. Drug Discovery Today, 2021, 26(1): 80-93. DOI: https://doi.org/10.1016/j.drudis.2020.10.010
- Jiménez-Luna J.; Grisoni F.; Weskamp N.; et al. Artificial intelligence in drug discovery: recent advances and future perspectives. Expert Opin. Drug Discovery, 2021, 16(9): 949-959. DOI: https://doi.org/10.1080/17460441.2021.1909567
- Friese A.; Ursu A.; Hochheimer A.; et al. The convergence of stem cell technologies and phenotypic drug discovery. Cell Chem. Biol., 2019, 26(8): 1050-1066. DOI: https://doi.org/10.1016/j.chembiol.2019.05.007
- Iwata H.; Kojima R.; Okuno Y. An in silico approach for integrating phenotypic and target-based approaches in drug discovery. Mol. Inf., 2020, 39(1/2): e1900096. DOI: https://doi.org/10.1002/minf.201900096
- Aulner N.; Danckaert A.; Ihm J.; et al. Next-generation phenotypic screening in early drug discovery for infectious diseases. Trends Parasitol., 2019, 35(7): 559-570. DOI: https://doi.org/10.1016/j.pt.2019.05.004